In my daily work, I’m becoming quite familiar with the ins and outs of using System.Text.Json. For those unfamiliar with this library, it was released along with .NET Core 3.0 as an in-the-box JSON serialisation library. At its release, System.Text.Json was pretty basic in its feature set, designed primarily for ASP.NET Core scenarios to handle […]

How Does the StringBuilder Work in .NET? (Part 3)
Part Three: How Appending Works and the StringBuilder Expands So far in this series, we’ve learned when we should consider using StringBuilder in our code and learned about the memory overhead of using a StringBuilder. It’s now time to learn how the StringBuilder can “expand” its capacity and support appending string data efficiently. As with […]

How Does the StringBuilder Work in .NET? (Part 2)
Part Two: Understanding the Overhead of a StringBuilder To continue exploring how the StringBuilder works, we’ll shift focus and study its logical design. Today, we’ll start by looking at how the type is designed and the overhead involved with creating and using StringBuilder instances. If you missed part one of this series, I explained why […]

How Does the StringBuilder Work in .NET? (Part 1)
Part 1: Why do we need a StringBuilder and when should we use one? After becoming proficient in .NET and C#, developers are likely to learn that they should use a StringBuilder to optimise string manipulation and concatenation. This is not a hard and fast rule for all situations but is generally good advice if […]
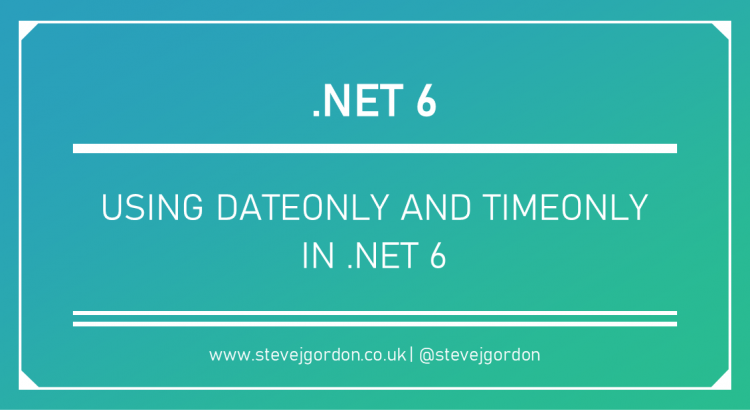
Using DateOnly and TimeOnly in .NET 6
In .NET 6 (preview 4), two long-awaited types have been introduced as part of the core library. DateOnly and TimeOnly allow developers to represent either the date or time portion of a DateTime. These two new types are structs (value types) and may be used when your code deals with date or time concepts independently. […]